This paper takes WC-12Co cemented carbide as the research object. Firstly, nano WC-Co composite powders were prepared by in-situ reduction carbonization reaction at different temperatures, and the composite powders were rapidly densified by discharge plasma sintering technology. The sintered blocks were systematically studied to analyze the effect of the in-situ reduction carbonization reaction temperature on the distribution characteristics of special crystal planes.
Experiment
The raw materials used in the experiment were carbon black, blue tungsten (purity 99.5wt.%, average particle size 50μm) and cobalt oxide (purity 98.5wt.%, average particle size 35μm). Carbon black, blue tungsten, and cobalt oxide were weighed according to the proportion for generating WC-12Co powder, and high-energy ball milling was carried out in a hard alloy ball mill jar with ethanol as the ball milling medium. The mixed powders were subjected to in-situ reduction carbonization reaction at 850°C, 900°C, and 1000°C in a vacuum environment, with a holding time of 1 hour. 2wt% of grain growth inhibitor was added to the composite powders prepared by in-situ reaction, and discharge plasma sintering densification was carried out. The sintering temperature was 1080°C, the holding time was 5 minutes, and the sintering pressure was 60MPa.
Material phase analysis was carried out on a Rigaku D/max-3c X-ray diffractometer with an acceleration voltage of 35kV and a current of 30mA, and a scanning rate of 2°/min. The micro-morphology of the powder was observed on a FEI-NovaNano SEM field emission scanning electron microscope.
Results and of Crystal Plane Distribution
Figure 1 shows the thermogravimetric (TG-DSC) curves of the nano WC-Co composite powder synthesized by in-situ reaction when heated to 1000°C at a heating rate of 10°C/min under Ar gas protection. From the change in the TG curve, it can be seen that as the temperature increases, the mass of the in-situ synthesized WC-Co composite powder decreases. When the temperature reaches 1000°C, the reaction ends, and the powder weight loss reaches nearly 6%. Throughout the heating process, the mass loss of the composite powder is divided into two stages: the first stage is from room temperature to 190°C, where there is a significant decrease in powder mass due to the ease of gas adsorption by nanoscale powders, and the gas desorbs and releases during the heating process, causing powder weight loss; the second stage is from 628°C to 1000°C, where the weight loss rate decreases from high to low. This is because the composite powder did not fully react during the low-temperature in-situ reduction carbonization reaction, and a secondary reaction occurred during the heating process, resulting in rapid weight loss of the powder.
Figure 2 shows the nanoscale WC-Co composite powder prepared by in-situ reduction carbonization reaction at 850°C. The powder particle size is mainly distributed between 30~120nm, with an average particle size of ~83.4nm. The particles have a good sphericity, and spherical or quasi-spherical powders exhibit good dispersibility and flowability, which can effectively avoid hard agglomeration of the powder. This is beneficial for the uniform dispersion of the powder in the sintering mold, thereby ensuring the uniformity and density of the sintered bulk structure. The composite powder prepared by the in-situ reduction carbonization reaction is subjected to rapid sintering densification in an SPS system to obtain nearly fully dense WC-Co cemented carbide blocks. XRD tests are conducted on the alloy specimens in the direction perpendicular to the sintering pressure and in the direction parallel to the sintering pressure. The relative integral intensities (i.e., integral area) of the diffraction peaks for each crystal plane in the VD (vertical to the sintering pressure) and PD (parallel to the sintering pressure) planes of the specimens are obtained through fitting calculations and compared with the relative intensities (integral area) of the diffraction peaks for each crystal plane in the PDF card (which represents the diffraction peak intensity distribution characteristics of traditional sintered cemented carbides), as shown in Table 1. The results indicate that the sintered WC-Co cemented carbide block specimens exhibit a significant characteristic of high anisotropic distribution for specific crystal planes.
Figure 3 shows the XRD patterns of the VD (vertical to the sintering pressure) and PD (parallel to the sintering pressure) planes of the specimens obtained by SPS sintering of the nanoscale WC-Co composite powder prepared by in-situ reaction at 850°C. From the figure, it can be observed that on the PD plane, the intensity of the (0001) plane peak is lower, while the intensity of the (10-10) plane peak is relatively higher. By fitting and calculating the integral area of each diffraction peak, the relative integral area of each crystal plane and the proportion of each crystal plane’s integral area in the total integral area are obtained, as shown in Figures 5 and 6.
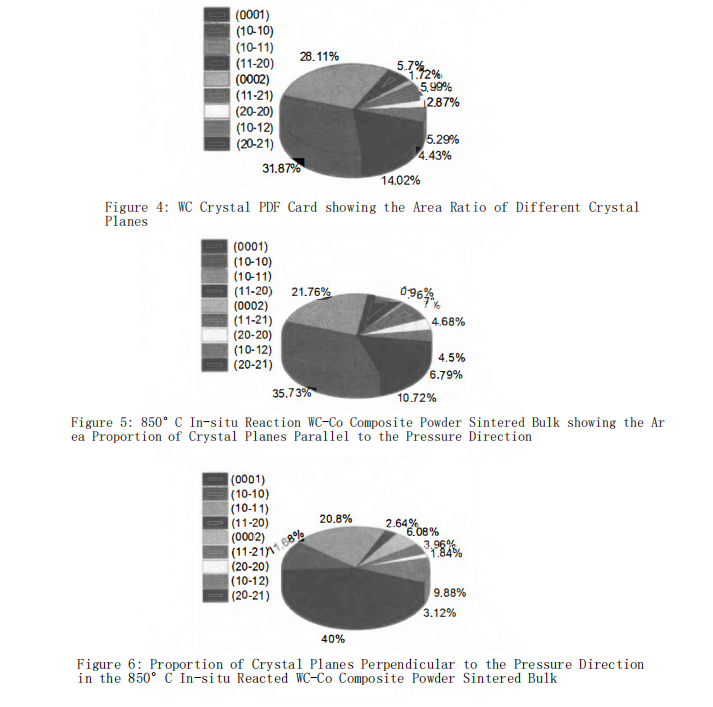
From Figure 5, it can be seen that on the PD plane, the integral area of the (0001) plane accounts for only 10.72% of the total integral area, which is a decrease of 3.3% from the 14.02% in the PDF card (as shown in Figure 4). The proportion of the (10-10) plane reaches 35.73%, which is higher than the 31.87% in the PDF card, and the proportion of the (10-11) plane decreases to 21.76% compared to the 28.11% in the PDF card. Compared to conventional cemented carbide samples, in the PD direction of the composite powder sintered block prepared by in-situ reaction at 850°C, the distribution proportion of the main characteristic planes (0001) and (10-11) decreases, while the distribution proportion of (10-10) increases.
Discussion
On the VD direction of the sample, as shown in Figure 6, the proportion of the (0001) crystal plane has significantly increased to 40%, compared to the 14.02% in the PDF card, while the proportion of the (10-10) crystal plane has decreased to 11.68%, a reduction of 20.19% from the 31.87% in the PDF card. From the above analysis, it can be understood that in the sintered block of composite powder prepared by in-situ reaction at 850°C, there is an orientation distribution of characteristic crystal planes. In the direction perpendicular to the pressure, WC grains rotate, causing the (0001) plane to become perpendicular to the pressure direction, thereby reducing the interfacial energy between WC grains. The sintered block of low-temperature in-situ reaction synthesis powder exhibits an orientation distribution characteristic of WC grain characteristic crystal planes, which is believed to be due to the formation of WC grains through secondary reactions during sintering, with atoms preferentially aligning along the (0001) plane and the rotation of WC grains under sintering pressure causing the (0001) plane to tend towards being perpendicular to the pressure direction.
When the temperature is raised to 900°C for in-situ reaction, the prepared composite powder is mainly WC with only a small amount of carbon-deficient phase. When the temperature is raised to 1000°C for in-situ reaction, pure WC-Co composite powder can be obtained. Since the crystal plane distribution of the grains in the powder is isotropic, the crystal plane distribution of WC grains does not change during the subsequent sintering densification process at 900°C and 1000°C, and they still exhibit a randomly distributed isotropic characteristic.
Fazit
1) The nanoscale WC-Co composite powder prepared by low-temperature in-situ reduction carbonization reaction at 850°C can be sintered into cemented carbide block materials with a highly oriented distribution characteristic of WC grain crystal planes using SPS. The sintered blocks of in-situ reaction powder at 900°C and 1000°C maintain an isotropic distribution of WC grain characteristic crystal planes.
2) In the WC-Co cemented carbide with highly oriented characteristic crystal planes, the basal plane (0001) occupies the largest area fraction in the direction perpendicular to the sintering pressure, reaching 40.0%; the prism plane (10-10) occupies the largest area fraction in the direction parallel to the pressure, reaching 35.7%.
3) The phase purity of the composite powder generated by the in-situ reaction plays an important role in the crystal plane orientation of the sintered block. When the main phase of the composite powder is WC, the crystal plane distribution of the sintered block does not exhibit oriented characteristics. However, when the main phase of the composite powder is the carbon-deficient phase, the crystal plane of the sintered block presents an oriented distribution characteristic.